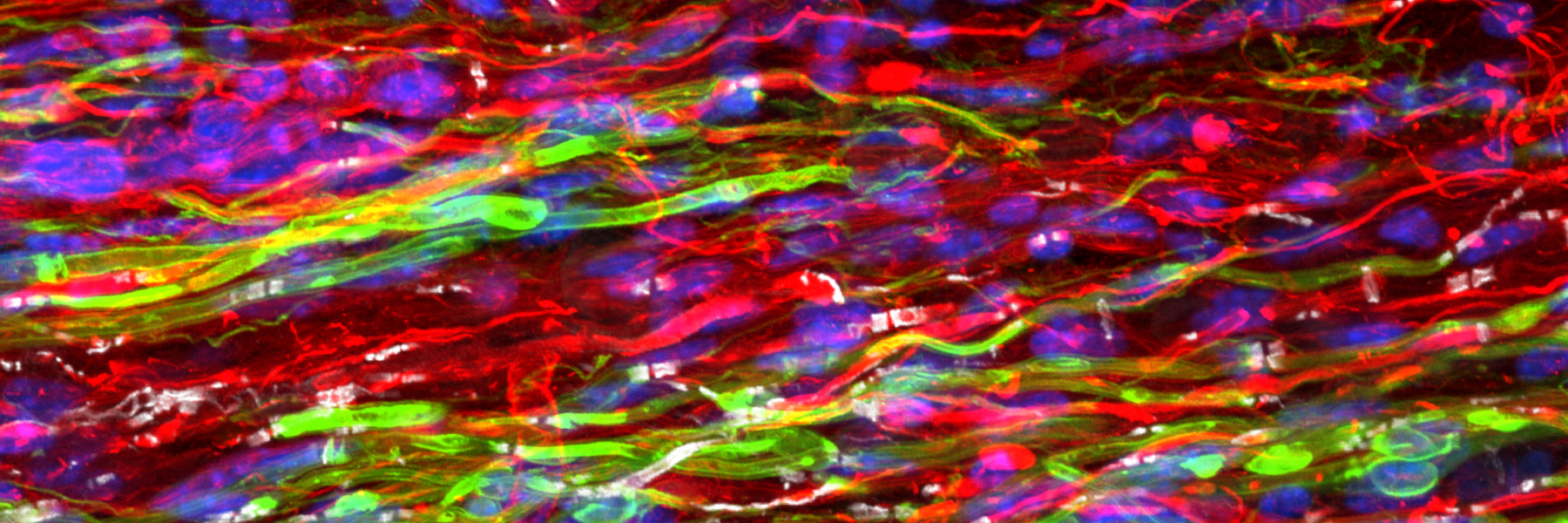
Current Projects
Bridging the Gap:
A major issue with traumatic damage to the CNS is the loss of cells. Regeneration of damaged tissue happens slowly if at all in the brain and spinal cord, therefore using stem cells to replace lost neurons and glia is an enticing strategy. Recently, our lab and others have demonstrated impressive engraftment and survivability of stem cells into the spinal cord and new technologies allow us to demonstrate impressive growth of a variety of cell types as well as long term survivability of newly formed neurons and glia from neural stem cell grafts. Despite these advances, better engraftment of complex stem cells has resulted in limited functional improvements. We leverage advanced inducible organoids created from human derived neural stem cells into complex structures before engraftment. Our strategy is two-fold where we pre-organize neural stem cells of the appropriate type into functional circuits with proper structure and organization prior to implantation and provide an electrical stimulation cue to improve overall growth and guidance. We believe that together, these two strategies will lead to more functionally organized and directed engraftment and improvement of upper limb function. Stem cell engraftments and electrical stimulation are both exciting technologies for the treatment of spinal cord injury. In the short term our goal is to target upper limb function, however, should the approach prove effective, there could be wide reaching clinical implications for many types of neural injury.
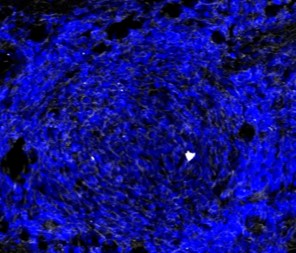
Wireless Spinal Stimulation:
Neural activity is an important modulator of plasticity. Many researchers are already harnessing the power of spinal stimulation to improve function after spinal cord injury. Our lab has developed a wireless stimulator designed to safely stimulate specific motor-associated circuitry in the spinal cord without using penetrating wires or other highly invasive methods. We have discovered that by placing this versatile system in different positions around the spinal cord, we can dramatically alter the overall response within the spinal cord. We received an R01 to examine the different responses of circuits to electrical stimulation. Using our state-of-the-art stimulation system, we hope to uncover new ways to position or improve existing spinal stimulation systems to enhance neural plasticity and improve outcomes after spinal cord injury.
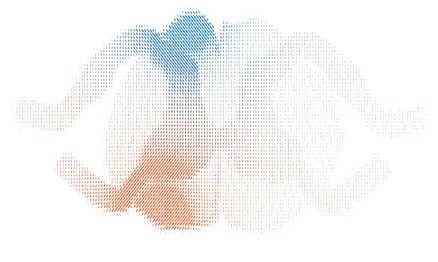
Neural Ribbon for Stem Cell Transplantation after SCI:
Neural stem cell (NCS) transplantation represents a forefront potential strategy to promote tissue repair after spinal cord injury (SCI). However, the uncontrolled NSC differentiation and the possible tumor formation after transplant remain a few of the main challenges. In Horner Lab, we engraft pre-differentiated neural stem cells specific for the location of SCI to increase robust long-term viability and functional improvement. Our innovative research consists in the transplant of cervical spinal motor neurons (SMNs) and interneurons that match the region of the cervical injury, instead of undifferentiated stem cells, plus oligodendrocyte precursor cells (OPCs) to restore the damaged neural circuitries. Moreover, to increase cell delivery efficiency, survival, and integration, we generated ex-vivo predefined neuronal networks in alginate hydrogel ribbons that we implant directly at the injury site in rat model of SCI.
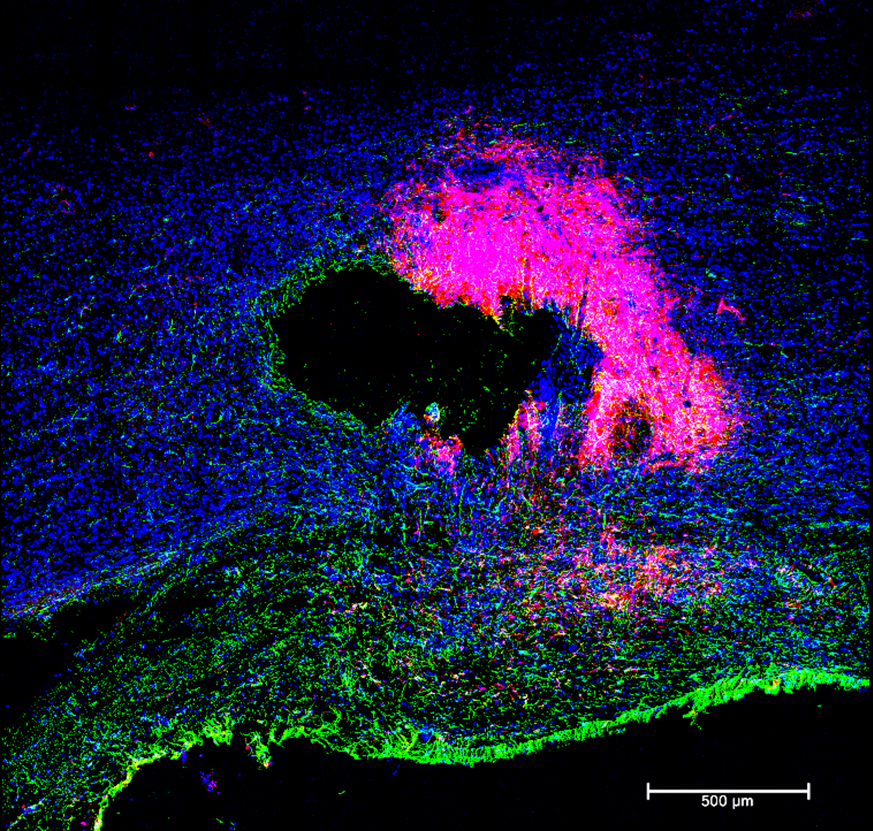
Immunomodulation and electrostimulation to promote regenerative microenvironment after SCI:
Spinal Cord Injury (SCI) is a complex condition where the loss of neural cells at the site of injury is associated with incomplete tissue repair and deficient neural regeneration. In Horner lab, we seek to develop an innovative therapeutic approach that addresses multiple issues associated with spinal cord injury and promotes an effective regenerative microenvironment. Our research shows that neuromodulation in the setting of SCI activates key gene regulatory networks that encourage functional recovery, in parallel with the suppression of the detrimental inflammatory processes in rat model of SCI. In light of our exciting findings, we propose to associate the tested electrostimulation protocol with our immunomodulation therapy consisting of the delivery of anti-inflammatory drugs specifically to inflammatory macrophages at the lesion site. The aim of this project is to create a comprehensive therapy that combines the immune toning of neuromodulation with the local drug delivery at the injury site to induce a natural repair cycle of the nervous system.
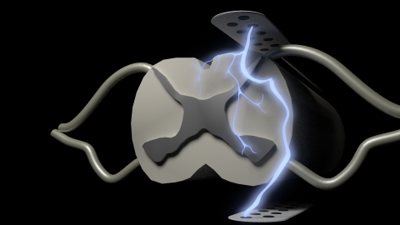
Microglia: A Stimulating Healer:
Microglia are the main immune cell in the CNS and are responsible for both coordinating the initial inflammatory response after injury and partaking in the long-term recovery process. Using gene expression analyses with our wireless spinal stimulators, we discovered that microglia may be playing a larger role in spinal cord repair than previously hypothesized. We hypothesize that neurostimulation drives microglia to an anti-inflammatory phenotype and thus dampens the neuroinflammatory response after spinal injury. This can potentially lead to an increase in spared synaptic connections and overall improving muscle function. Our goal is to apply bioinformatic techniques and use gene expression and epigenetic analysis to better understand how microglia behave in response to injury and neurostimulation. We believe that this approach will bring forth many microglial pathways that are exploitable for improving spinal injury recovery and functional outcomes in patients.
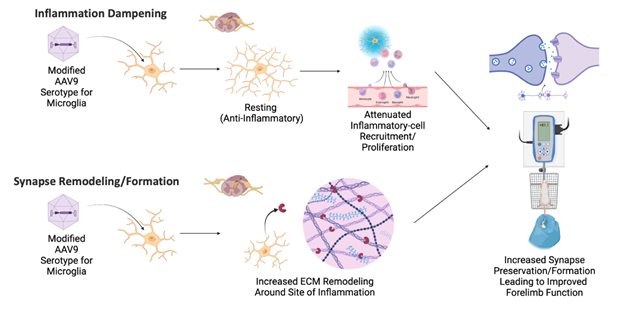
Behavioral Assessment following SCI:
Cervical spinal cord injuries (SCI) are amongst the most severe spinal injuries affecting humans due to drastically impacting quality of life through the impairment of stereotyped movements and highly integrated sensorimotor functions, resulting in a high fatality rate. Other than physical therapy, which can only assist in gaining partial function, there is currently no treatment providing full recovery for cervical SCI. At the Horner Lab, rat models have been implemented to assess motor learning, control of fine movements, and track progression of recovery after cervical SCI through the forelimb reaching task (FRT). Additionally, the lab has been implementing the limb-use asymmetry test (LUAT), which assists in determining the limb-preference pre and post cervical SCI, as well as throughout the short and long-term recovery periods. Overall, the behavioral assessments of the rats demonstrate the degree of injury observed after a cervical SCI, allowing us to learn more about the natural progression and effects of the injury to implement therapeutic strategies that could improve quality of life.
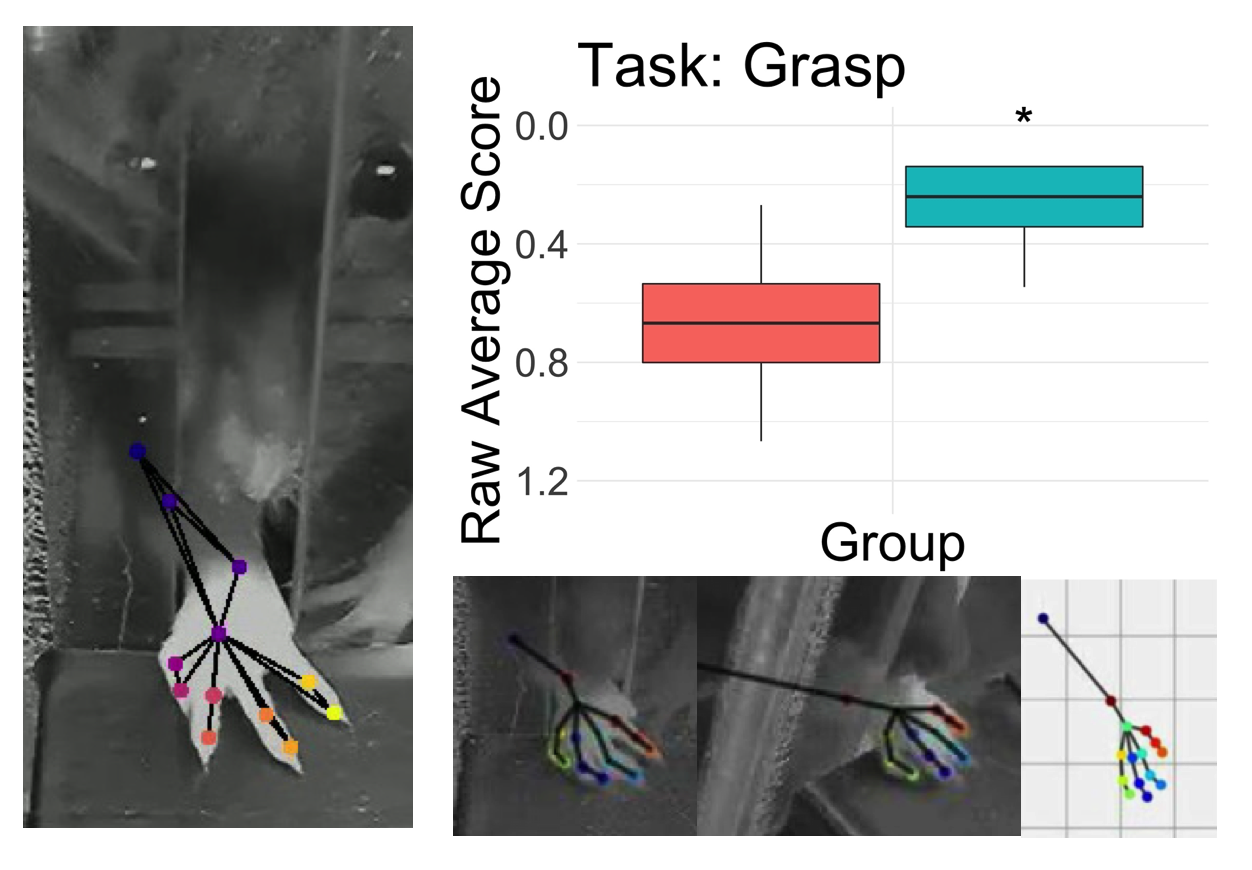
Injectable Gel for Spinal Injury:
We have developed a two part gel which can be injected straight into a neural injury zone. After spinal cord injury, a scar forms around the injury zone and neurons and other cells retract and die leaving an empty non-functional space in the spinal cord. The scar around the space is formed by a cell called astrocytes and it is rubbery and impenetrable to healthy neurons. Our healing gel fills empty space left after injury and is designed to promote neural growth back into the injury zone. It also slowly releases a drug to soften the scar and allow regeneration. We believe this two part approach is a powerful tool to combine with other strategies to treat spinal cord injury.

Optogenetics – Light to control neurons:
Light to control neurons: In order to discover how to manipulate neural activity to heal the spinal cord, we need to understand how different firing patterns influence neural growth and behavior. Using a gene adapted from algae, researchers have made neurons that fire in response to specific colors of light. We can now grow human neurons with this Channelrhodopsin gene and rapidly mature them using a built in genetic switch. Not only are we able to control their activity patterns with pulsed blue light, we can also ask questions in a matter of days rather than in the months it takes other researchers in the field. This powerful technology allows us to define how human neurons respond to different stimulation patterns. These patterns could possibly be adapted for spinal or brain stimulation therapy to improve outcomes after spinal trauma or even stroke.
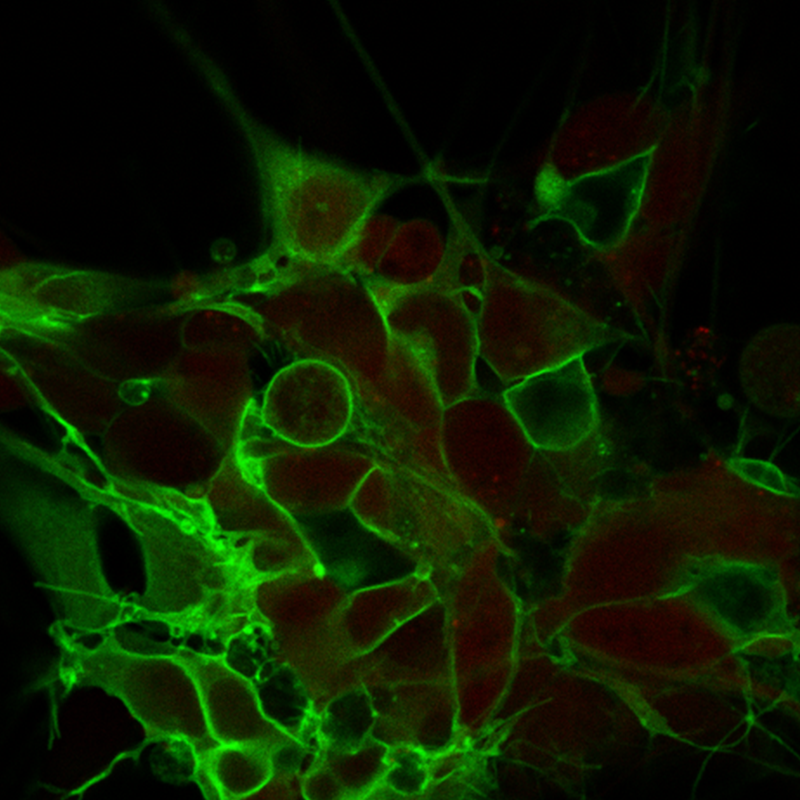
The Stroke Model:
Using pig stroke models, we study different candidates influencing neural cell fate and neural plasticity, the effects of activity on gene expression in the injured spinal cord, and virtual reality processing/analysis of 3D medical/research data.
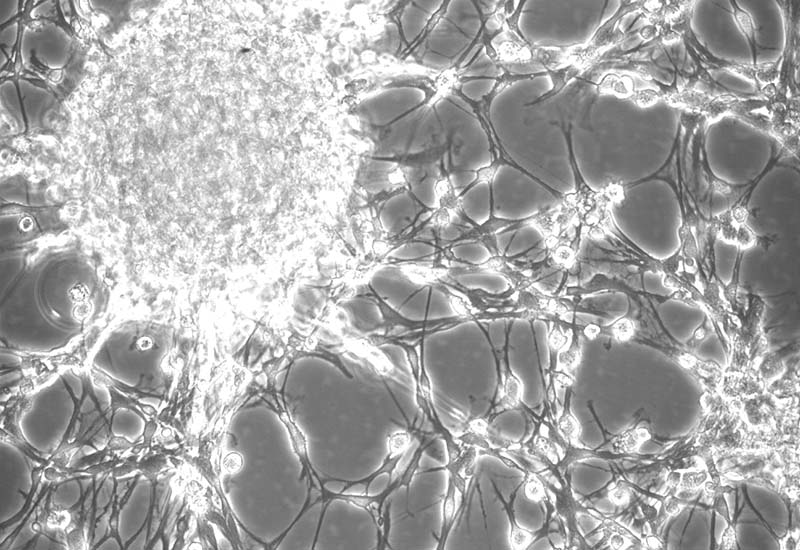
Unlocking the Mechanisms of Myelin Plasticity:
The Horner lab has previously used a retrovirus coupled to a membrane bound mouse reporter to differentiate between old and new myelin after spinal cord injury. This unique tool will be the key to unlocking the mechanisms of myelin plasticity and regeneration.
1) Using this tool as a myelin labeling reporter, we can distinguish distinct myelin stages and implement profiling studies to identify novel genes involved in regeneration and plasticity.
2) We are also building a retroviral toolbox to easily perform gain- and loss-of-function studies. The retrovirus can then be used to label developmental myelin and follow plasticity over time, or after injury to distinguished function in regenerating myelin.
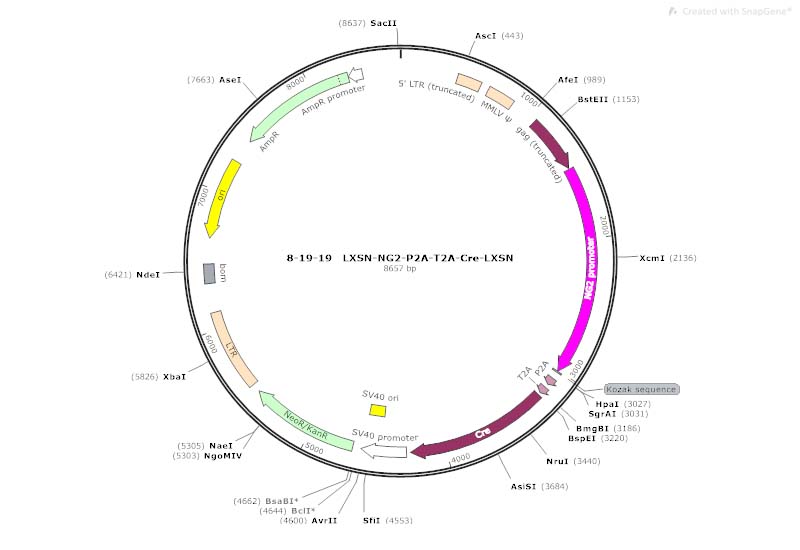
Activity Dependent White Matter Plasticity Following Traumatic Neural Injury:
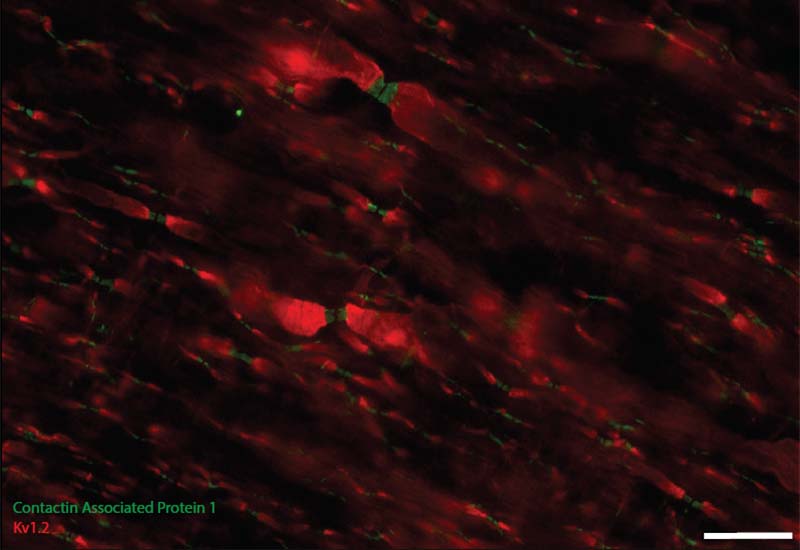
Staining for contactin associated protein 1 (CASPR) allows us to visualize node of Ranvier re-formation following injury. Scale bar = 25 microns.
Brain Stimulation Trial for Neuroprotection Following Stroke:
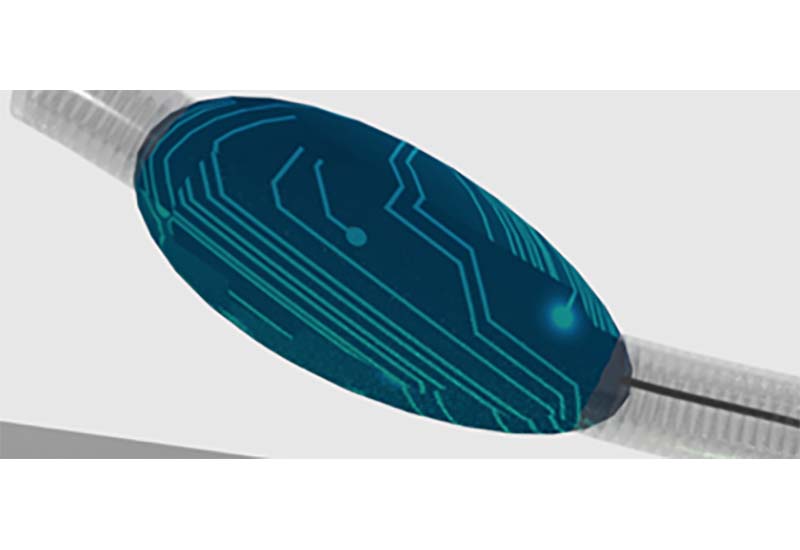